Building a mechanobiological signalling scaffold for the heart
A collaboration between scientists at the Mechanobiology Institute (MBI), National University of Singapore and clinical researchers from the Cardiovascular Research Institute (CVRI), National University Healthcare Systems, revealed how a scaffolding protein integrates biochemical and mechanical signals to control cardiac muscle cell differentiation.
We all know how muscles lose definition when we don’t exercise for a period of time. Fortunately, most of our muscles have the ability to renew and regenerate from muscle stem cells, so getting back into shape can be done with exercise and effort. However, certain muscles have a limited regenerative potential once fully developed, such as the heart, despite the essential role it plays in constantly pumping blood around the body. As damage accumulates over time from lifestyle factors, disease, or ageing, the heart will become progressively weaker and eventually fail.
Therefore, many researchers have sought to understand the mechanism by which muscle cells in the heart differentiate into specialised cardiac muscle to see if there may be a way to enhance cardiac muscle growth and regeneration. One of the major challenges in this revolves around the unique function of the heart. Going back to the example of exercise we can see how the heart needs to interpret both biochemical and mechanical signals. For instance, during a strenuous workout the heart may need to respond to a sudden rush of adrenalin hormones – a biochemical signal – by increasing the mechanical rate at which the heart is beating. The reverse needs to occur once the workout is finished.
To investigate the workings of the heart, an interdisciplinary team of scientists from the Mechanobiology Institute joined forces with clinicians from the Cardiovascular Research Institute, NUHS. The research team was headed up by MBI Research Scientist (and ex-MBI graduate student), Dr. Darren Wong, under the supervision of MBI Principal Investigators Assoc. Prof. Low Boon Chuan (Department of Biological Sciences and NUS College) and Assoc. Prof. Pakorn Kanchanawong (Department of Biomedical Engineering) in partnership with consultant cardiologist Prof. Roger Foo (NUS Yong Yoo Lin School of Medicine). This interdisciplinary study used a combination of genetic and biochemical approaches in conjunction with biophysical analysis and super-resolution microscopy imaging.
Given the integrative nature of the heart, the team focused on the signalling scaffold protein BNIP-2. As the name suggests, scaffold proteins work by bringing together multiple proteins so they can interact. This scaffolding is achieved through a specific region on BNIP-2 termed the ‘BCH domain’. The BCH domain is conserved, meaning it can commonly be found on other proteins. Previous work from the Low lab has shown that the BCH domains target members of the Rho protein family that act as molecular switches to regulate signalling pathways, and BNIP-2 scaffolding has been implicated in cell movement, growth, and skeletal muscle cell signalling.
An initial genetic experiment revealed that increased expression of heart genes involved in force generation (i.e. those coding for cardiac muscle proteins that power heart contraction) correlated with increased expression of the BNIP-2 gene and protein. Conversely, inhibition of BNIP-2 expression resulted in alteration in various cardiac signalling pathways, specifically activation of targets of the YAP protein signalling pathway. YAP is a well-known mechanosensitive protein that moves in and out of the nucleus in response to mechanical signals. When YAP is in the nucleus, it typically helps to turn on genes that promote cell and tissue growth by increasing cell number and size. These sets of “growth-promoting” genes will be turned off when YAP is sequestered out of the nucleus in the cytoplasm.
In this case, the research team discovered that when the cardiac muscle genes were switched on, YAP was inactivated and prevented from entering the nucleus. Using super-resolution microscopy, they observed that the scaffold function of BNIP-2 enables it to interact with a protein called LATS1, which binds to and sequesters YAP outside of the nucleus. Based on the mechanosensitive nature of YAP, the researchers then investigated the role of contractile force, as regularly experienced in the heart, on cardiac muscle gene differentiation. They found that contractile force was required for the expression of cardiac muscle genes, and remarkably BNIP-2 also plays a key role in this process. By measuring traction force generation and the activity of RhoA, they discovered that BNIP-2 indeed activates RhoA – a molecular switch that regulates the force-generating machinery within the heart cell.
‘Forcing’ the building of a heart
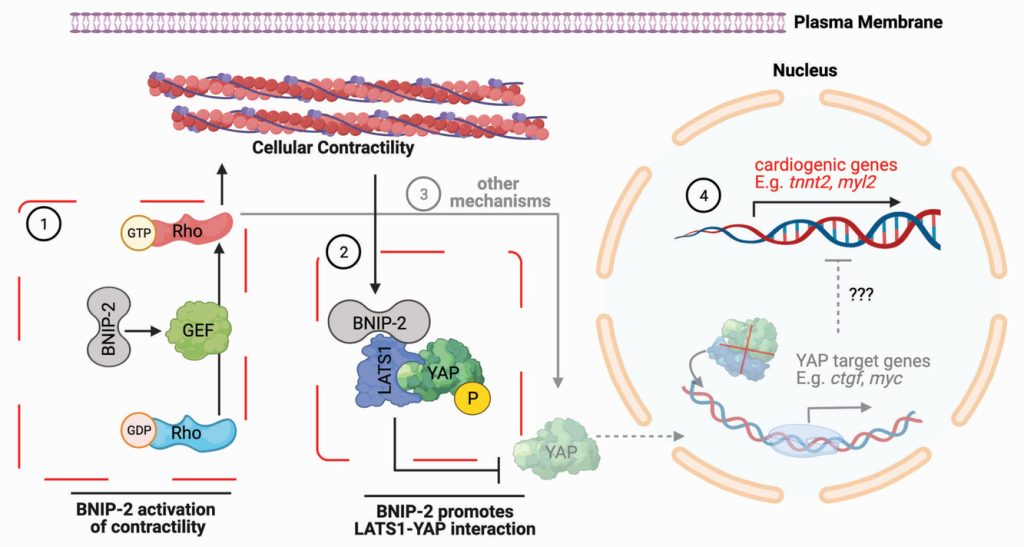
Figure 1: Schematic showing the dual roles of BNIP-2 inactivation of YAP for cardiac muscle differentiation
Taken together, the team revealed the multifaceted role of BNIP-2 in driving cardiac muscle differentiation. BNIP-2 activates the RhoA molecular switch, which turns on the force-generating machinery leading to cell contractility. This mechanical signal promotes the scaffolding interaction between BNIP-2 to LATS1, which in turn binds to YAP. This biochemical signalling pathway results in YAP being sequestered out of the nucleus, resulting in growth genes turning off and induction of cardiac muscle genes. This finding is the first step towards understanding how mechanical and biochemical signals work together to control cardiac muscle differentiation.
This successful collaboration between MBI and CVRI will continue as a part of a larger Ministry of Education Tier 3 research programme aimed at investigating the molecular and mechanobiological underpinnings of cardiac ageing. Perhaps even more roles for BNIP-2 in the heart remain to be discovered!