Microtubules and Cell Movement
A Closer Look at Focal Adhesion Disassembly
Researchers from the Bershadsky Lab at MBI utilized optogenetics to unlock the role of microtubules in regulating focal adhesion disassembly, an important step in cell migration. This study was published in The EMBO Journal; https://doi.org/10.1038/s44318-024-00114-4
Writer: Justin Lu. Editor: Andrew Wong.
The cytoskeleton is a component of all eukaryotes, bacteria, and archaea, which acts as a dynamic skeleton, blood vessels, and nerves for the cell. It consists of actin filaments, intermediate filaments, and microtubules. Microtubules are hollow rods in the cytoskeleton that serve as a mobile set of bones for the cell, helping maintain cell structure and allowing intracellular transport and cell division. Another critical function of microtubules is interacting with focal adhesions to regulate cell polarity and help with cell migration. In a study from the 1970s, complete disruption of microtubules using the drug colchicine was shown to stop directional cell migration. Focal adhesions are the points on the outer cell membrane that make contact with the outside environment of the cell, i.e., the extracellular matrix or substrate. They are made up of many proteins that connect integrins (receptors used by the cell to bind to extracellular surfaces) to the intracellular actin filaments.
While focal adhesions are necessary for cell migration, too stable and long-living adhesions can interfere with this process. Microtubules are known to promote focal adhesion turnover, thereby promoting cell migration. This is because the cell moves by attaching new focal adhesions and releasing the focal adhesions at the rear end of the cell as it goes. Without this important disassembly process, the cell would be stuck in place and attached to the substrate by focal adhesions. Despite their importance, the exact mechanisms through which microtubules influence focal adhesions have remained unclear until now.
To unravel these mysteries, a collaborative team of researchers from the Mechanobiology Institute at the National University of Singapore from the lab of Prof. Alexander Bershadsky, led by Research Fellow Julien Aureille, decided to investigate how this process is orchestrated. They focused on KANK family proteins, which dynamically link microtubule tips with focal adhesions. To observe the linkage between microtubules and focal adhesions, the researchers developed an innovative optogenetic construct that they dubbed OptoKANK, with which they could control the linkage using blue light. This novel approach allowed the researchers to capture the natural environment and various naturally occurring processes within cells and observe the result in real time.
The team then found that when they activated OptoKANK using blue light, microtubules would converge on individual focal adhesions and quickly retract, causing them to slide and disintegrate due to a lack of tensile force. The process was found to be dependent on myosin-II, which is a key protein that generates contractile forces. The study showed that myosin-II built up near the focal adhesion, leading to a rise in traction force and, ultimately, the disassembly of the focal adhesions. Furthermore, the scientists discovered that the activator protein GEF-H1, commonly associated with microtubules, was essential for this focal adhesion disintegration. GEF-H1 activates the Rho signaling pathway, which leads to an increase in myosin filament assembly. Inhibition of GEF-H1 eliminated the generation of traction force from myosin, preventing sliding and disassembly of the focal adhesions.
The team found that for reasons unknown, as the microtubules detach from the focal adhesion, the dormant GEF-H1 is released from the microtubules and becomes active, triggering Rho proteins and increasing myosin filament levels. Without contractile force generation from this myosin and the subsequent disassembly of the focal adhesions, cell migration would not be possible, and the cell would be stuck in place, trapped by the focal adhesions on the rear end of the cell.
Along with their experimental findings, the team collaborated with Professor Alex Mogilner of NYU to develop a mathematical model to depict this novel microtubule-driven focal adhesion disassembly process. The model simulated the number of microtubules attached to and the amount of KANK molecules located on an individual focal adhesion, and the density of related proteins with proximity to the focal adhesion. This model incorporated the local GEF-H1-dependent activation of contractility and was consistent with their experimental data, including various alterations of the experiments. It provided a quantitative framework for understanding how microtubule targeting leads to the rapid disassembly of focal adhesions.
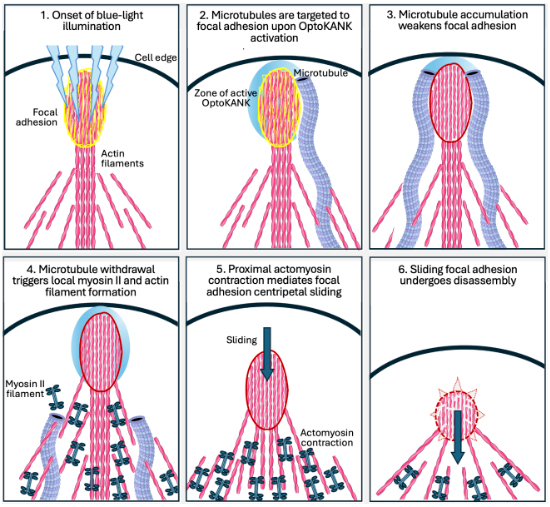
Progressive microtubule capture during focal adhesion maturation ultimately triggers focal adhesion turnover. Graphical abstract from https://doi.org/10.1038/s44318-024-00114-4
By revealing that focal adhesion disassembly is driven by the localized activation of contractile forces, this study provided valuable insights into cellular dynamics. More specifically, the team discovered a new function of microtubules as a sensor to activate GEF-H1 when attached to adhesions. This finding has broader implications in cell migration as this new function of microtubules could be applied to other areas. Additionally, the new knowledge of cell migration is essential for understanding critical processes like wound healing, immune response, and cancer metastasis. The team’s innovative use of optogenetics to manipulate cellular structures, a technique that allows scientists to study cellular processes with high spatial and temporal precision, also presents a powerful tool for future research, opening new avenues for exploring the complex interplay between different cellular components.